Biological Physics
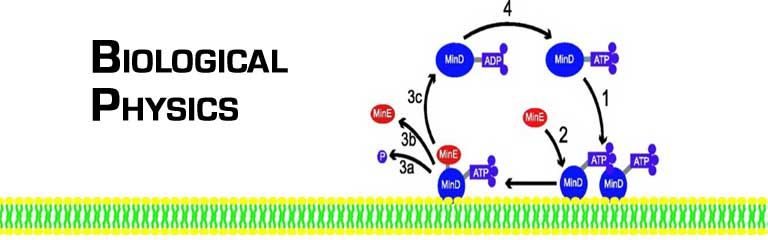
Biological functions of cell membranes are controlled by the dynamic organization and interactions of the membrane's main building blocks, lipid and proteins. For simplicity, synthetic lipid membranes are usually studied as mimics of cell membranes in order to tease out reliable information of the interplay between membrane composition, structure, and dynamics and the resultant membrane functions. Using a suite of techniques including x-ray and neutron scattering and MD simulations, the aim of this project is to understand:
- Nanoscale membrane structure
- Effect of membrane composition on domain formation/structure and interactions
- Fast membrane dynamics and their role in biological functions
- Membrane bending and thickness fluctuations
- Membrane protein interactions
This project aims to investigate curvature effects in surface-modulated lipid membranes. This is done by utilizing the tunability of nanopatterned thermoresponsive polymer scaffolds to generate controllable 2D architectures in supported lipid membranes. The premise of this system is that it enables real-time realization of lateral membrane reorganization and peripheral protein binding in response to local membrane curvature, thus providing insights into critical membrane functions such as signal transduction, cell trafficking, and host-pathogen interactions. Further, such tunability of membrane topography can open new avenues to thermally switchable membrane-based biosensors.
Polymer nanocomposites (PNCs) are promising candidates for advanced multifunctional light-weight materials. The premise of PNCs lies in the myriad of possibilities they offer in synergistically integrating particle and polymer properties to obtain substantially improved material performance. Although earlier research on nanocomposites has remarkably enhanced our understanding of PNCs, less explored phenomena such as individual and collective chain dynamics and thermodynamically-driven wetting and dispersion phenomena, currently pose significant challenges to the applicability of PNCs in next-generation technologies. The focus of this project is to combine x-ray/neutron scattering with spectroscopy and simulations to understand the hierarchy of structures and dynamics and design advanced PNCs with controlled material properties.
Research interests in Professor Täuber's group are in soft condensed matter and non-equilibrium systems. Specific research interests include: structural phase transitions; dynamic critical behavior near equilibrium phase transitions; phase transitions and scaling in systems far from equilibrium; statistical mechanics of flux lines in superconductors; and applications of statistical physics to biological problems. The group employs Monte Carlo and Langevin molecular dynamics simulations to solve stochastic equations of motion, as well as field theory representations to construct perturbational treatments and renormalization group approaches that improve on mean-field approximations.
Prof. Kaplan's research interests are in theoretical soft matter and biological physics. In close connection to experiments, his group develops theories and simulations to elucidate the interplay between the material composition, dynamics, form, and emergent function in living systems and their synthetic analogs.