Experimental Condensed Matter Physics
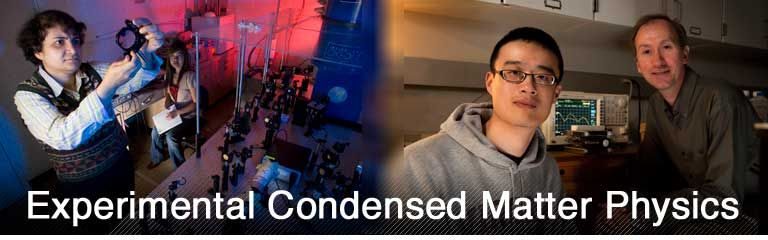
Faculty: Rana Ashkar; Satoru Emori; J. R. Heflin; J. J. Heremans; G. Khodaparast; V. Nguyen; H. Robinson; V. Soghomonian
Emeriti Faculty: A. L. Ritter; R. Zallen
Prof. Emori’s research interests are in nanometer-thick materials with robust spin-driven physics. Many of the physical phenomena studied are pronounced at room temperature and are essential for next-generation computing and communications technologies. Examples include low-loss spin dynamics in epitaxial oxide thin films, spin torque effects arising from thin-film interfaces, and chiral domain walls in ultrathin heterostructures. The group’s experimental capabilities encompass synthesis of high-quality magnetic thin films and heterostructures, tabletop measurements of spin transport and dynamics, and element-specific static and dynamic magnetic characterization at synchrotron facilities.
Biological functions of cell membranes are controlled by the dynamic organization and interactions of the membrane's main building blocks, lipid and proteins. For simplicity, synthetic lipid membranes are usually studied as mimics of cell membranes in order to tease out reliable information of the interplay between membrane composition, structure, and dynamics and the resultant membrane functions. Using a suite of techniques including x-ray and neutron scattering and MD simulations, the aim of this project is to understand:
- nanoscale membrane structure
- effect of membrane composition on domain formation/structure and interactions
- fast membrane dynamics and their role in biological functions
- membrane bending and thickness fluctuations
- membrane protein interactions
This project aims to investigate curvature effects in surface-modulated lipid membranes. This is done by utilizing the tunability of nanopatterned thermoresponsive polymer scaffolds to generate controllable 2D architectures in supported lipid membranes. The premise of this system is that it enables real-time realization of lateral membrane reorganization and peripheral protein binding in response to local membrane curvature, thus providing insights into critical membrane functions such as signal transduction, cell trafficking, and host-pathogen interactions. Further, such tunability of membrane topography can open new avenues to thermally switchable membrane-based biosensors.
Polymer nanocomposites (PNCs) are promising candidates for advanced multifunctional light-weight materials. The premise of PNCs lies in the myriad of possibilities they offer in synergistically integrating particle and polymer properties to obtain substantially improved material performance. Although earlier research on nanocomposites has remarkably enhanced our understanding of PNCs, less explored phenomena such as individual and collective chain dynamics and thermodynamically-driven wetting and dispersion phenomena, currently pose significant challenges to the applicability of PNCs in next-generation technologies. The focus of this project is to combine x-ray/neutron scattering with spectroscopy and simulations to understand the hierarchy of structures and dynamics and design advanced PNCs with controlled material properties.
Heflin's research group focuses on organic self-assembled optoelectronic nanostructures and devices. Areas of investigation include ionic self-assembled multilayers for polar second order nonlinear optics, nanoscale control of composition in organic photovoltaics comprising semiconducting polymers and fullerenes, biosensors based on self-assembled films on optical fiber long period gratings, anti-reflection coatings via nanoparticle self- assembly, and patterned self-assembly for nanoscale electronic devices. This effort is part of the Center for Self-Assembled Nano- structures and Devices.
Heremans' laboratory studies the physics of controllably fabricated semiconductor, metal and organic nanostructures, through their electronic and magnetic properties. Areas of study include spin electronics and spin-dependent, quantum-coherent electronic transport in nano-patterned semiconductor heterostructures; electronic transport in molecular systems; electronic transport in organic semiconductor structures; and magnetic sensor geometries on high-mobility semi- conductors.
The lab utilizes in-house nanoscale fabrication techniques such as electron beam and scanning probe lithographies, and various patterning and deposition techniques. Measurements occur at typically very low excitation levels, low temperatures (0.3 K), and under magnetic fields (up to ∼10 T). Apart from the fabrication equipment, the lab houses measurement cryostats, characterization equipment (SEM, AFM, profilometer, optical microscopes), and equipment for sensitive electronic measurements.
Khodaparast's research activities are focused on understanding the quantum states and charge/spin dynamics in low-dimensional systems. She uses magneto-optical and time-resolved ultrafast spectroscopy to study materials such as semiconductor hetero- structures, magnetic semiconductors, and quantum dots.
These research activities will develop concepts for new devices as well as elucidate fundamental physics. The experimental facilities utilized include a femtosecond near-infrared laser, a parametric optical amplifier, and cryogenic equipments.
Nguyen's group works both in experimental condensed and soft matter physics. In particular, the group is interested in the dynamics of electrons confined in very tiny semiconductor structures and dynamics of biological molecules through Terahertz spectroscopy.
Robinson's group combines high sensitivity optical and electrical measurement techniques to investigate the physics of semiconducting and metallic nanostructures.
Projects include investigations of optically manipulated single electron spins and spin currents in reduced-dimensional nanostructures at low temperatures, and the use of localized plasmonic resonances on metallic nano- structures to enhance non-linear effects such as second harmonic generation, Raman scattering, and the electro-optic effect.
Soghomonian's group focuses on the synthesis, fabrication and characterization of biological, organic, and hybrid organic/inorganic nano-scale devices and assemblies.
Areas of study include charge transport measurements through variously modified DNA molecules to elucidate the relationship of DNA structure to its charge transport properties, charge transport measurements in organic and bio-organic single crystal and thin film geometries to elucidate charge injection characteristics in these systems, atomic force microscopy studies of self-assembled patterns of biomolecules on various surfaces, and hydro- and solvo-thermal synthesis of novel zeolitic materials with desired functionalities.